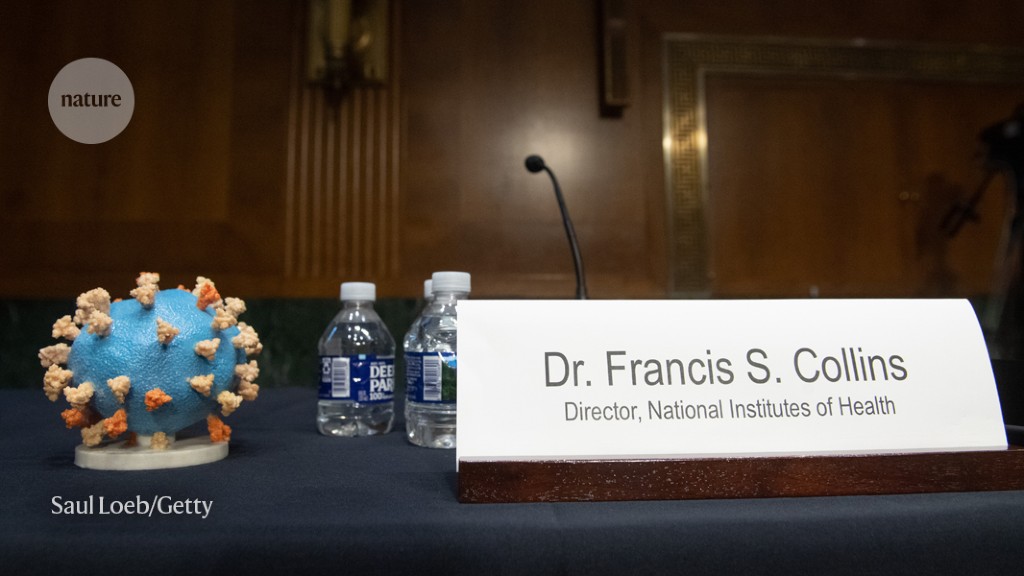
Four lessons from the pandemic to reboot the NIH - Nature.com
From innovation to workforce diversity, scientists are eager for change at the world’s largest funder of biomedical research.
From innovation to workforce diversity, scientists are eager for change at the world’s largest funder of biomedical research.